The integrated single mode laser converter is an efficient, simple device that relies on the principle of Raman laser conversion for generating a single longitudinal mode (SLM) or a single-frequency laser output. A SLM laser operates on a single resonator mode, so that it emits quasi-monochromatic light with a very narrow linewidth and very low phase noise. Single longitudinal mode diode lasers have a much narrower bandwidth, making them more desirable for applications that require precise knowledge of the wavelength.
Qubik Photon is a CERN spin-off that intends to manufacture and sell a low-cost single longitudinal mode efficient laser. The applications for this technology is in diverse areas such as spectroscopy, LIDAR (light or laser imaging, detection and ranging), optical metrology, communication and quantum applications for singular light sources.
Explore the technology specifics:
Qubik Photon is a CERN spin-off that intends to manufacture and sell a low-cost single longitudinal mode efficient laser. The applications for this technology is diverse and we wish for startups to build applications based on this novel laser tech. This tech can make any cheapo multimode high power laser into a tunable and efficient single longitudinal mode laser.
Source: https://www.rpmclasers.com/blog/laser-diode-fundamentals-what-are-longitudinal-modes/#:~:text=A laser is classified as,only one mode to lase. Laser diodes must meet two critical requirements to facilitate the lasing process. The first requires that, at a minimum, the laser cavity’s gain increases to the point that it reaches unity with the level of losses. This unity state is known as the gain threshold. The second requirement is that there must be a longitudinal mode present inside the optical cavity, coinciding with the laser’s gain curve. All lasers must contain three key components: an active gain medium, a resonator cavity, and an excitation/pump source. To understand what longitudinal mode is and how it’s derived, we will focus solely on the resonator cavity, ignoring the gain medium and excitation/pump source. To further clarify our initial analysis, we will also assume that both mirrors in the resonator cavity are 100% reflective, effectively giving us what’s known as an “ideal resonator.” In the simplest case, the laser’s optical cavity is formed by two opposed plane (flat) mirrors surrounding the gain medium (a plane-parallel or Fabry–Pérot cavity). In this scenario, all light waves are trapped within the cavity, creating a standing wave as the light propagates and reflects between the two mirrors. For a better conceptualization, think of how guitar strings can sustain the same note or frequency, resulting from the wave propagating along the medium and reflecting off the string’s fixed ends (standing waves).
What is a Laser? Similarly, an infinite number of standing waves can exist inside this resonator cavity, but they all must have a node at the two mirrors. The allowed modes of the cavity are those where the mirror separation distance L is equal to an exact multiple of half the wavelength, λ:
where q is an integer known as the mode order. Therefore,
a the wavelength or frequency of these standing waves is governed by the resonator’s length, with the lowest order mode having a wavelength of one half the cavity length and each higher-order mode have a wavelength equal to ½ , where n is the mode number. A simple formula shows that each mode in the cavity will be spaced out according to the relationship ,
called the free spectral range, where c is the speed of light, n is the index of refraction, and L is the cavity length. Given this quantization of suitable frequencies/wavelengths in the laser resonator cavity, we can see that an infinite number of modes can fit into the resonator, all with equal frequency spacing. These Fabry-Perot modes, as they’re called, heavily influence the laser’s spectral characteristics. However, no mirror is 100% reflective, and if they were, you couldn’t use them to generate a laser beam. A cavity with 100% reflective mirrors would block all transmission of light, making it impossible to output a usable beam. Since all practical resonator cavities will exhibit some loss at the mirrors, the Fabry-Perot modes can not be pure delta functions; rather, they have an inherent line width that varies based on their reflectivity percentage. A detailed analysis of the causes of this line broadening is beyond the scope of this blog post. However, the figure below illustrates the effects mirror reflectivity has on the mode structure. You can acquire the specific, allowable spectral modes by superimposing the laser diode’s gain band on top of the Fabry-Perot cavity modes, as shown in the figure to the right. The laser can only produce these remaining modes (or wavelengths), referred to as the laser’s longitudinal modes. If a particular longitudinal mode’s gain is greater than the total cavity loss (the gain threshold), amplification of that mode is allowed, and lasing can occur. The interrelationship between gain threshold, gain bandwidth, and longitudinal mode spacing ultimately determines whether a diode operates as a single or multi-mode device. A laser is classified as ‘single longitudinal mode‘ (SLM) when the gain threshold is significantly high, and the gain bandwidth substantially narrow, allowing for only one mode to lase. Conversely, a laser is classified as ‘multi-longitudinal mode‘ (MLM) when the gain band is substantially broad and the threshold significantly low, allowing for many modes to lase at once. Both multi-longitudinal mode and single longitudinal mode diode lasers have their inherent pros and cons. Multi-longitudinal mode diode lasers are typically more straightforward and cost-effective to produce, with the added benefit of generating much higher output powers, due to the larger number of modes. On the other hand, single longitudinal mode diode lasers have a much narrower bandwidth, making them more desirable for applications that require precise knowledge of the wavelength. However, these diode lasers typically produce much lower power and are more challenging to manufacture.
- A single-frequency laser (or single longitudinal mode laser) is a laser that operates on a single resonator mode, so that it emits quasi-monochromatic light with a very narrow linewidth and very low phase noise. As a direct consequence, they exhibit a very long coherence length of greater than 10 meters and up to 1000 meters, which makes them suitable for long-range communications and detection applications. Typically, the excited mode is a Gaussian mode, and so the output is diffraction limited. The area of application of single-frequency lasers covers mainly spectroscopy, optical metrology(e.g. with fiber-optic sensors) and interferometry. Other applications include optical data storage, LIDAR, holography, Raman spectrometry, semiconductor inspection, coherent remote sensing, laser communication, or Laser Doppler velocimetry, etc. All these applications require very narrow spectrum and long coherence length lasers. Unfortunately, most single frequency lasers in the market are either low power, not tunable or very expensive and complex. Singular Light is a project that solves all these problems at once. By exploiting non-linear optical phenomena (such as Raman processes), our solution is capable of converting conventional inexpensive high power multi-mode lasers into a tunable single longitudinal mode efficiently, easily and at low cost. Moreover, our device is power scalable. Integrated Single Mode Laser Converter, is an efficient, simple device that relies on Raman laser conversion for generating a single longitudinal mode (SLM) laser output. The configuration offered by Singular Light not only offers a more robust generation of SLM lasers compared to conventional techniques, but it also allows ease of adjustment of the output laser frequency. These characteristics make the technology a perfect fit to answer the requirements of many areas of optical metrology and interferometry, from data storage to optical communications. With the advent of diamond quantum computing, Singular Light also presents a unique opportunity for the integration of compact laser sources on a diamond photonic chip.
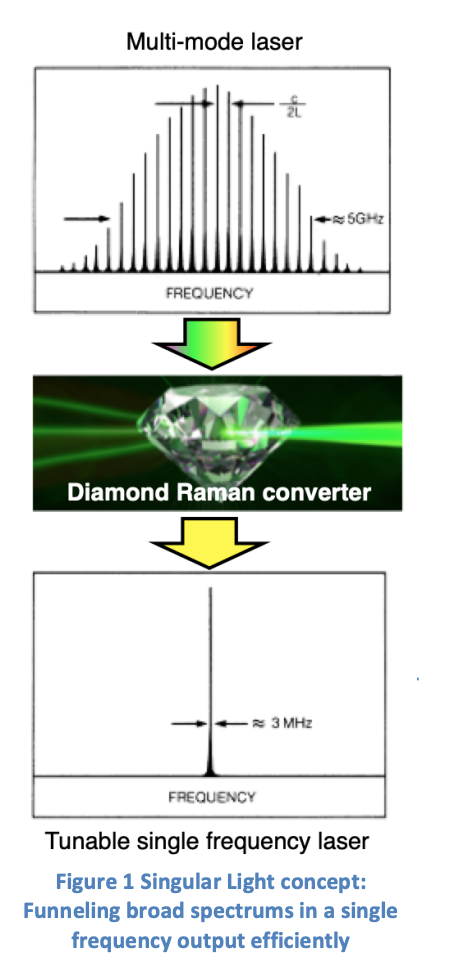
- Demonstration of simultaneous multi-colour output from the Raman converter, measured high quality beams
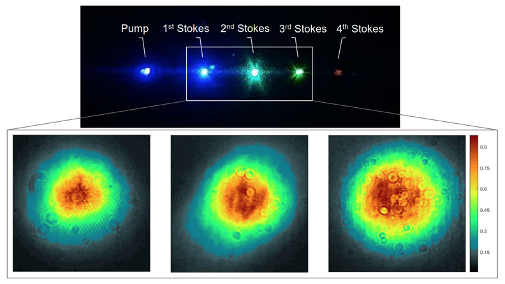
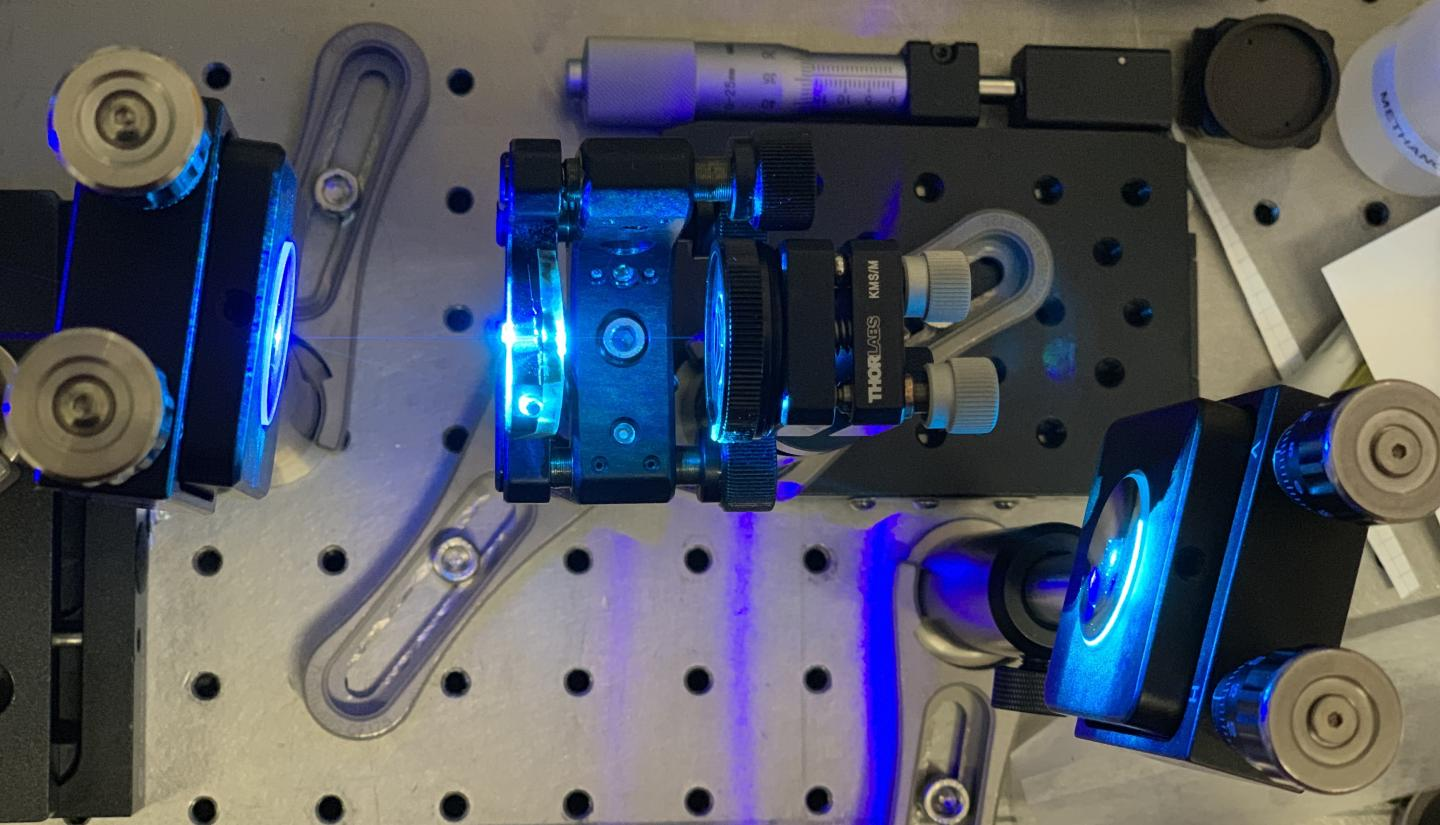
- Efficient generation of a single longitudinal mode (SLM) laser with a very small linewidth and low phase noise, already demonstrated an enhancement of 100x of peak power spectral density with > 40% average power conversion efficiency. Laser output exhibits a rather long coherence length (from 10 up to 1000 metres), with a Fourier limited output of ~100 MHz linewidth.
- Frequency-shifted laser output, with respect to the laser pump input by 30 - 50 nm, providing access to new wavelengths.
- Ease of adjustment of the output laser frequency via length tuning of monolithic diamond resonator via angle or temperature.
- Extremely simple optical configuration, compared to conventional methods of generating SLM lasers Resilient against mode competition thanks to spectral hole burning free Raman gain technology.
- Robust to external disturbances, thanks to complete integration of laser into a diamond host and no need for free-space optical components.
- Single mode in both the longitudinal and transversal direction.
The technology is unique because of its ofering.
Special features +
- Low-cost
- Extremely compact spot size and very low divergence
- Non-diffractive over long distances
- Self-constructive after obstacles
- Robust to jitter, vibrations, and variations on the angle of the input beam
The technology can have many applications in:
- Quantum applications for singular light sources
- Spectroscopy.
- LIDAR
- Optical metrology
- Communication
Patent :
- https://worldwide.espacenet.com/patent/search/family/074180919/publication/WO2022129604A1?q=pn%3DWO2022129604A1
- https://www.qubikphoton.com/projects-6
- https://opg.optica.org/ol/abstract.cfm?uri=ol-47-16-3976
- https://opg.optica.org/optica/fulltext.cfm?uri=optica-9-3-317&id=470556
- https://opg.optica.org/ol/abstract.cfm?uri=ol-47-9-2210